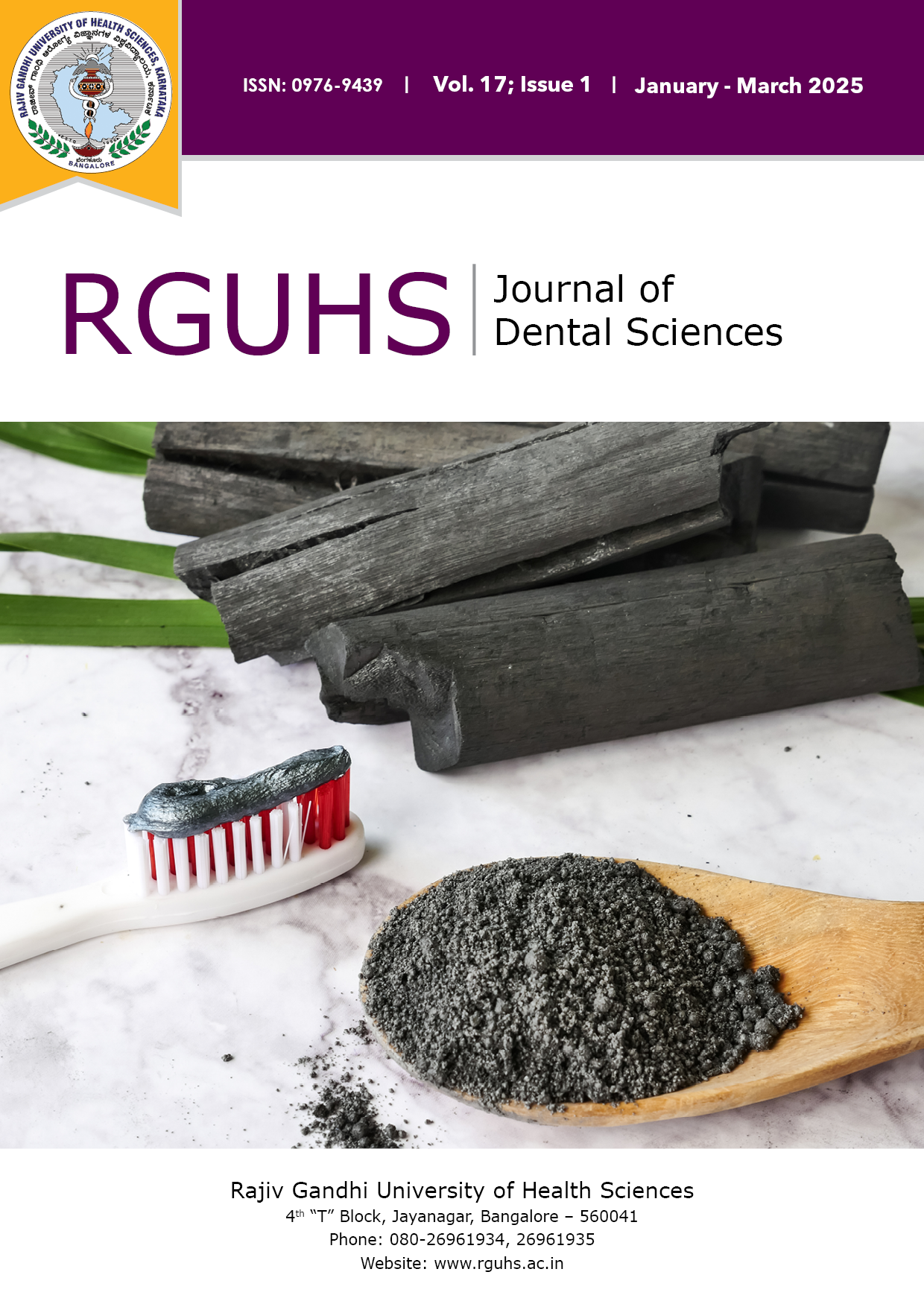
RGUHS Nat. J. Pub. Heal. Sci Vol No: 17 Issue No: 1 pISSN:
Dear Authors,
We invite you to watch this comprehensive video guide on the process of submitting your article online. This video will provide you with step-by-step instructions to ensure a smooth and successful submission.
Thank you for your attention and cooperation.
Varshitha KV*, SP Pushpa, Nidagundi N
Department of Periodontology, Maratha Mandal’s Nathajirao G Halgekar Institute of Dental Sciences and Research Centre, Belagavi, Karnataka, India.
*Corresponding author:
Dr. K.V.Varshitha, Department of Periodontology, Maratha Mandal’s Nathajirao G Halgekar Institute of Dental Sciences and Research Centre, Belagavi, Karnataka, India. E-mail: varshireddy72@gmail.com
Received date: 4/01/22; Accepted date: 24/02/22; Published date: 30/09/2022

Abstract
Dentistry is a continuously evolving science. Pathogenic oral biofilms are universal and chronic which leads to the destruction of the tissues due to the presence of the micro-organisms in its matrix. Treatment of bio-film-associated infections requires the development of new therapeutic strategies. In recent years, new nano-technology-based antimicrobials have been designed. Nanoparticle-mediated eradication uses the activation of the catalytic iron oxide nanoparticles (CAT-NP) coated with dextran (Dex-NZM). It results in the disruption of the biofilm by killing planktonic, antibiotic-resistant bacteria. It causes synergistic, antibiofilm efficacy which lowers the potential for bacterial resistance by free radical production, degradation of bacterial components, and causing bacterial cell death. Hence, the present study enumerates an alternative newer approach to the complete eradication and further prevention of oral biofilms.
Keywords
Downloads
-
1FullTextPDF
Article
Introduction
Nanotechnology is employed in many aspects of our daily lives, including medicine. It is simple to study and modifies atoms, chemical bonds, and molecules present between diverse substances using nanotechnology. The term “nanodentistry” refers to the application of nanotechnology in the dental field.1 Catalytic nanoparticles have been shown to disturb biofilms and have gained attention in the dental fraternity. Because of their inherent qualities, nanomaterials may be used as a delivery vehicle for transportation of the drugs to the infected site, or they may be used as active therapeutic agents.2 The interactions between biofilm and therapeutics are important for determining the treatment outcome. These antimicrobial nanoparticles either coat the biofilm surfaces or stop biofilm development thereby preventing its formation.3 Treating a pre-existing biofilm, on the other hand, is far more challenging. To completely eradicate biofilms, antimicrobial treatments must penetrate and accumulate within the biofilm. Nanoparticles can be designed to promote drug solubility and could be delivered into bacterial cells for their antibacterial action. Metal or metal oxides, hybrids, and synthetic or natural polymers can all be used to make antibiofilm nanoparticles.4
Gram-negative bacteria are more susceptible to nanoparticles compared to gram-positive bacteria. Negatively charged lipopolysaccharide molecules coat gram-negative bacteria. These negatively charged molecules have a stronger affinity for positive ions that most nanoparticles release, resulting in ion accumulation and absorption, which causes intracellular damage.5
The following are the key mechanisms that explain nanoparticles’ antimicrobial effects: 1) bacterial cell membrane rupture; 2) production of reactive oxygen species (ROS); 3) bacterial cell membrane penetration; and 4) development of intracellular antibacterial effects, including interactions with proteins and DNA.6
Dextran is a microorganism-derived polysaccharide that may be integrated into the matrix by bacterial exoenzymes (e.g., Streptococcus mutans-derived glucosyltransferases) that use dextran as an acceptor molecule to generate Exopolysaccharides (EPS) glucans when given to a developing biofilm.7 The Food and Drug Administration (FDA) has approved dextran-coated iron oxide nanoparticles (Dex-NZM) which are available as Feridex, for systemic uses such as contrast agents in Magnetic Resonance Imaging (MRI) scans.8 Dextran coating would result in aqueous formulation stability as well as biocompatibility with the human soft tissue in the mouth. Dex-NZM was created using dextran with a variety of molecular weights ranging from 1.5 kiloDalton (kDa) to 40 kDa. To evaluate the best coating qualities, their catalytic properties, biofilm uptake, cytotoxic effect, and antibiofilm activity are taken into consideration. At a molecular weight of 10 kDa, dextrancoating provided maximum biofilm uptake, catalytic activity, and antibiofilm characteristics.9
In a study on rat model biofilms, no systemic adverse effects (e.g., no reduction in rat weight) were observed when iron oxide/hydrogen peroxide was applied topically for 21 days daily.9
Properties
Chemical nature and physical architecture such as size, shape, surface and functionalization dictate nanoparticle features while thecore qualities affect antibiofilm efficacy (for solid nanoparticles). (Figure 1)10
After topical treatment, nanoparticle size has an effect on diffusion into the EPS biofilm matrix, with diameters as small as 130 nm suggesting significant biofilm penetration.10
Positively (cationic) charged nanoparticles had better biofilm penetration than anionic or uncharged counterparts, possibly as a result of the catch-and-release procedure inside the anionic EPS matrix.11
In addition, the hydrophobic end of the cationic nanoparticles is taken up by the bacteria whereas the hydrophilic end gets linked to the EPS.11 The basic properties of nanoparticles (e.g., solid or hydrophilic/ hydrophobic depots) allow the administration of several antibiofilm agents. Core-shell nanoparticles with cationic and hydrophobic cores capable of loading antimicrobial oils demonstrate strong antibiofilm activity as well as selective cytotoxicity against bacteria compared to fibroblast cells.12
Spherical nanoparticles have the highest cellular uptake with little membrane damage, while rod- or other shaped nanoparticles have a larger contact area with cell membrane receptors, thus creating more membrane damage and possibly chronic inflammation.13
Silica particles, dendrimers, micelles, or liposomes can be loaded with antimicrobials, photosensitizers, or enzymes to either allow them to freely circulate in the blood without causing damage or better penetrate a biofilm. Drugs with poor aqueous solubility can be transported to infection sites. Triclosan, protoporphyrin IX, farnesol, and essential oils are hydrophobic antimicrobials that can be delivered as cargo in the hydrophobic core of micelles, whereas the hydrophilic core of many liposomes can be loaded with hydrophilic drugs.10
To guarantee correct nanoparticle localization and enhance oral antibiofilm activity, the size, charge, and core characteristics of the nanoparticles are used.14
The interplay between nanoparticle size and shape can be utilized to improve the efficacy of biofilm-targeting nanoparticles. It has not been confirmed as a critical design characteristic for oral biofilm therapies.14
Antibiofilm effect
When catalytic nanoparticles (CAT-NP) are combined with hydrogen peroxide, ROS is produced (Figure 2). The oxidation of macromolecules and cell components caused by ROS-mediated oxidative stress usually results in cell damage. This suggests that ROS has a direct role in the destruction of the biofilm EPS matrix and the death of S. mutans. In acidic pH conditions, iron oxide particles show significant peroxidase-like activity against S. mutans biofilms. Due to the pH-responsive activity of nanoparticles, free radical production is decreased under physiological circumstances, normal tissues are protected from off-target effects. In a study, despite the beneficial effect of CAT-NP on rat biofilm model by topical application, the colloidal stability of iron oxide particles was poor, and tissue binding was indiscriminate which limited overall therapeutic translation.15 As a result, Naha et al (2019) created Dex-NZM dextran coatings for CAT-NP, which preserved the underlying iron oxide catalytic activities while increasing the selective attachment of nanoparticles to biofilm compared to gingival tissues.9
Uses in dentistry1
Dental fillings
Polishing enamel surface
Antisensitivity agent
Tooth whitening
Prevention of caries Uses in periodontology
Antibacterial coatings on dental implants.
Targeted delivery to pathogenic bacteria.
It creates an environment of oxidative stress to kill periodontal pathogens.
Reduces bleeding by selective and controlled release of drugs into cells and tissues at periodontal inflammation sites.
Advantages:9
Site specificity Bio-compatible
Economical
Disadvantages:9
Peroxidase-like activity is seen only when pH is acidic. Tooth staining
Conclusion
Dextran-coated iron oxide nano-enzymes are effective antibiofilm agents. This approach could provide an excellent therapeutic platform to prevent periodontal diseases. Their adaptability and capacity to interact in multiple ways make them ideal targets for planktonic bacteria. In vivo studies revealed no adverse effects on the surrounding host tissues and oral microbiota diversity by histological and microbiome analyses. No cytotoxic effect and biofilm-targeting specificity were observed in vitro. The chronic consequences of routine treatment regimens, opportunities for enhanced efficacy, and novel biomimetic techniques should be carefully considered to ensure the continuous progression of oraland healthcare-associated biofilm nanoparticle therapies.
Conflict of Interest
None
Supporting File
References
1. Priyadarsini S, Mukherjee S, Mishra M. Nanoparticles used in dentistry: A review. J Oral Biol Craniofac Res 2018;;8(1):58-67.
2. Sharma V. K, Yngard R. A, Lin Y. Silver nanoparticles: green synthesis and their antimicrobial activities. Adv Colloid Interface Sci 2009,;145(1−2):83−96.
3. Hajipour MJ, Fromm KM, Ashkarran AA, et al. Antibacterial properties of nanoparticles. Trends in biotechnology. 2012;30(10):499-511. Trends Biotechnol 2012;30(10):499−511.
4. Benoit DS, Sims Jr KR, Fraser D. Nanoparticles for oral biofilm treatments. ACS Nano. 2019;13(5):4869- 75.
5. Slavin YN, Asnis J, Häfeli UO, et al. Metal nanoparticles: understanding the mechanisms behind antibacterial activity. J Nanobiotechnol 2017;15(1):1-20.
6. Wang L, Hu C, Shao L. The antimicrobial activity of nanoparticles: present situation and prospects for the future. Int J Nanomed 2017;12:1227. 7. Gibbons R J, Banghart S B. Synthesis of Extracellular Dextran by Cariogenic Bacteria and its Presence in Human Dental Plaque. Arch. Oral Biol 1967;12:11-23.
8. Corot C, Robert P, Idée JM, et al. Recent advances in iron oxide nanocrystal technology for medical imaging. Advanced drug delivery reviews.Adv. Drug Deliv. Rev 2006;58(14):1471-504
9. Naha PC, Liu Y, Hwang G, et al. Dextran-coated iron oxide nanoparticles as biomimetic catalysts for localized and pH-activated biofilm disruption. ACS Nano. 2019;;13(5):4960-71.
10. Liu Y, Shi L, Su L et al. Nanotechnology-Based Antimicrobials and Delivery Systems for BiofilmInfection Control. Chem. Soc. Rev. 2019;48(2):428- 46.
11. Li X, Yeh YC, Giri K, et al. Control of nanoparticle penetration into biofilms through surface design.. Chem. Commun. 2015;51(2):282-5.
12. Duncan B, Li X, Landis R F, et al. NanoparticleStabilized Capsules for the Treatment of Bacterial Biofilms. ACS Nano 25;9(8):7775-82
13. Sharifi S, Behzadi S, Laurent S, Forrest ML, Stroeve P, Mahmoudi M. Toxicity of nanomaterials. Chem Soc Rev. 2012;41(6):2323-43.
14. Slomberg DL, Lu Y, Broadnax AD, et al. Role of size and shape on biofilm eradication for nitric oxide-releasing silica nanoparticles. ACS Appl. Mater. Interfaces. 2013;5(19):9322-9.
15. Gao L, Liu Y, Kim D, et al. Nanocatalysts promote Streptococcus mutans biofilm matrix degradation and enhance bacterial killing to suppress dental caries in vivo. Biomaterials. 2016 Biomaterials 2016;101:272-84.
16. Cormode DP, Gao L, Koo H, et al. Emerging biomedical applications of enzyme-like catalytic nanomaterials. Trends Biotechnol 2018;36(1):15- 29