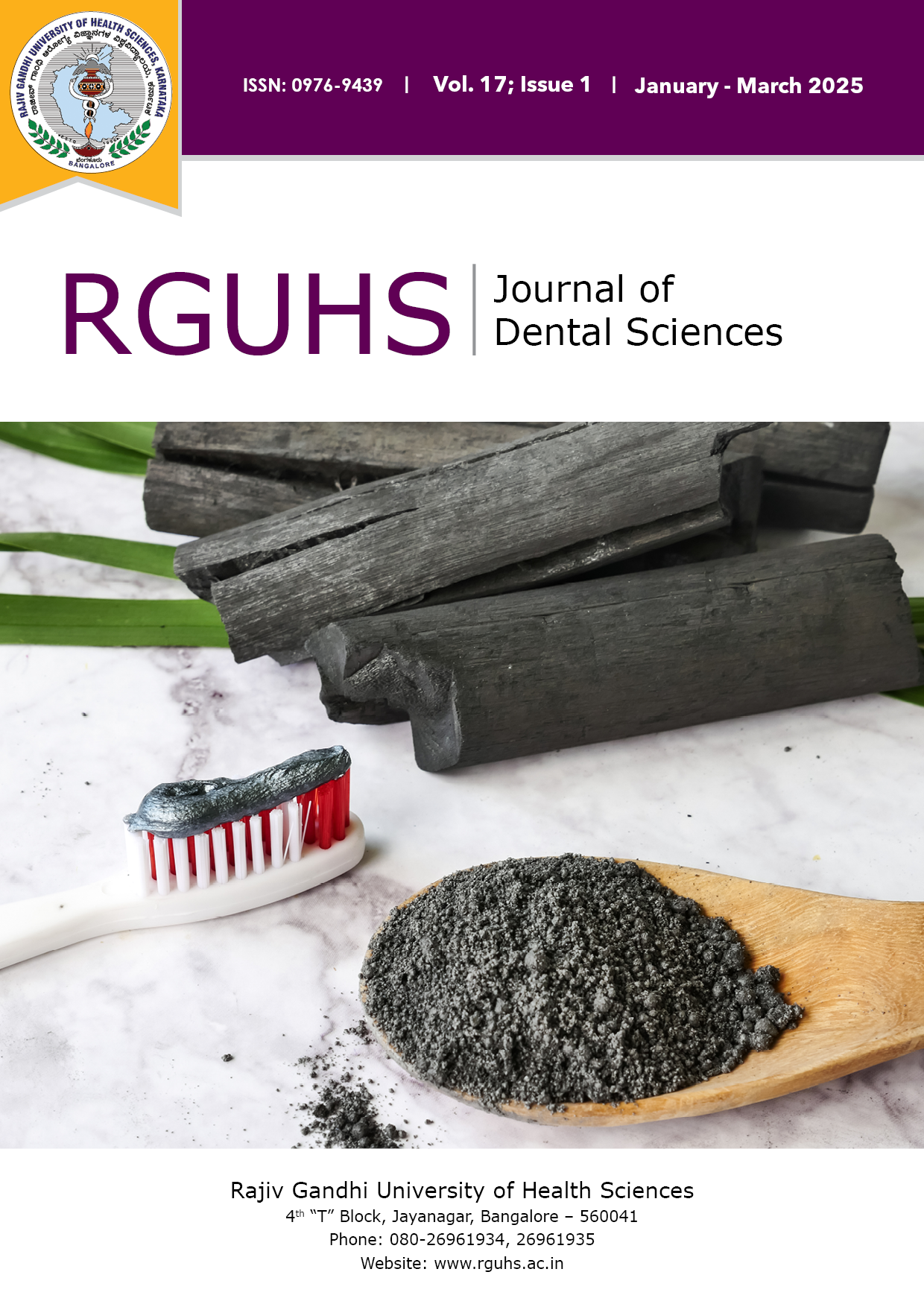
RGUHS Nat. J. Pub. Heal. Sci Vol No: 17 Issue No: 1 pISSN:
Dear Authors,
We invite you to watch this comprehensive video guide on the process of submitting your article online. This video will provide you with step-by-step instructions to ensure a smooth and successful submission.
Thank you for your attention and cooperation.
1Dr. Moitri Ojha, Senior Lecturer, Department of Periodontology, SMBT Dental College, Sangamner, Maharashtra, India.
2Department of Conservative Dentistry and Endodontics, SMBT Dental College, Sangamner, Maharashtra, India
3Department of Preventive and Pediatric Dentistry, Dental College, RIMS, Ranchi, India.
*Corresponding Author:
Dr. Moitri Ojha, Senior Lecturer, Department of Periodontology, SMBT Dental College, Sangamner, Maharashtra, India., Email: moitriojha@yahoo.in
Abstract
The current scenario of dentistry focuses on regeneration for bony defects and associated disorders. For this, a thorough understanding of biological and molecular sciences is required. Bone substitutes (natural or synthetic) act as fillers and scaffolds to regenerate bone and stimulate wound healing. A variety of bone grafts are used in dental practice, the most common being alloplasts (synthetic bone grafts). Globally, 2.2 million bone grafts are placed every year. However, there are several challenges yet to overcome. Modifications of existing bone grafts continues to be an expanding subject of interest. Poly (D,L-lactic co-glycolic) acid (PLGA) - a copolymer of lactic acid and glycolic acid is the rising star in regenerative science which is a complex field. This composite biomaterial retains the properties of both the monomers and can be modified and manipulated into desired forms. Initially, PLGA was a desirable candidate for carrier of drug, peptides and proteins delivery. Owing to the versatile properties of PLGA, it has currently encroached various pharmaceutics, medical and dental arenas. This material has been approved by US FDA (Food and drug administration) as a bone graft substitute. It is believed that PLGA scaffolds can generate osteoconductive and osteoinductive gradient useful for bone formation. This polymer offers the advantages of controlled degradation, biocompatibility, tunable physiochemical properties and ability to regenerate bone tissues. Also, it demonstrates low toxicity in biological tissues. More recently, it has revealed its potential as scaffolds, 3D printed platforms, hydrogels, fibers, microspheres and nanoparticles. This literature review elaborates the implications of PLGA in regeneration therapy.
Keywords
Downloads
-
1FullTextPDF
Article
Introduction
Since time immemorial, the unquenchable thirst of human race to discover the potential of new materials has led to the evolution of material science. Broadly, matter is classified into organic and inorganic components. Polymers are macromolecules that can be in either of these categories. Specifically, organic polymers laid the plinth of life on our earth. One among such many known polymers is Poly lactic co-glycolic acid (PLGA).
The unique properties of PLGA includes magnetic, mechanical, thermal, etc. Such attributes made this material applicable in various sectors like commercial, military and environmental. Currently it has gained popularity in the field of nano-medicine, pharmaceutics and imaging.1 Apart from its application in diagnosis, tissue engineering and drug delivery, it is now becoming an icon in bone regeneration.
PLGA can easily breakdown within our body – the hydrolysis of which leads to the production of lactic acid and glycolic acid. These two products of hydrolysis are common natural byproducts in many metabolic processes of our body that are eliminated by the renal system. Therefore, the biocompatibility of PLGA is remarkable.
Besides being a tissue-friendly material, it can be fabricated into many forms like sheets, ribbons, particles and fibers which can be of micro or nano configurations. Lactic and glycolic acid ratio can also be adjusted to achieve the desired degradation rate. Commercially, they are available in various ratios.
Bone graft substitutes are employed to repair and regenerate damaged bone tissues. These bone grafts are categorized depending on the method of procurement and mode of action (Table 1). The most widely used are alloplasts.2 Alloplasts are readily available in the market, demonstrates good handling properties, induces good osteoconductive environment and show reduced morbidity.3
The various forms of alloplasts are mentioned in the Table 2.
Literature review suggests that PLGA has good compressive strength and can be combined with various bone graft substitutes. Also, this polymer can be integrated with many growth-promoting factors and regenerative cells for an enhanced outcome.4 This integration of regenerative grafts with each other to derive an improved and desirable outcome is referred to as composite bone grafts as they combine the properties of osteoconduction, osteogenesis or osteoinduction. Studies have shown that PLGA bone composite possess suitable properties like biocompatibility, degradability and osteoconductive properties. Taking these facts into consideration, this literature review is an attempt to comprehend the enigma of PLGA in bone regeneration.
Conception and Evaluation
Homo and co polymers of lactic and glycolic acid have been explored since 1950’s, but its recognition and commercialization commenced after a decade. In 1966, Kulkarni et al. confirmed the non-toxic nature of PLA in surgical implants. Schmitt and Polistina, on the other hand, developed Polyglycolic acid (PGA) sutures with the commercial name “Dexon” in 1967. In 1970’s, PLGA was approved by FDA as suitable material for surgical sutures. Vicryl sutures (containing PLGA), established by Ethicon in 1974 gained popularity among clinicians and surgeons. After achieving this milestone, PLGA was researched in the area of controlled drug release and injectable microparticles.3 It was then considered as a “gold standard polymer” for controlled delivery system.5 In the year 1981, the first clinical human trial on PLGA particles was carried out. Decapeptyl was the first PLGA microspheres-based product that brought hopes for prostate cancer patients.6 Till date, more than 35 products of PLGA have been effectively launched in the market.
Formulation Considerations
PLGA is generally an acronym for poly (D,L-lactic-co-glycolic) acid where D- and L- lactic acid forms are in equal ratio. The degradability of PLGA is governed by the glycol units. Higher the number of glycol units, lesser is the time required for degradation and vice versa.7 Some formulations of the polymer tend to accumulate in liver, bone marrow, spleen, lymph nodes, etc. The clearance of the drug by the body is rapid and is effectively performed by kidneys and lungs.
PLGA can be modified to obtain the desired features in terms of hydrophilicity/lipophilicity and degradation rate. In fact, copolymers containing a high fraction of lactic acid are less hydrophilic, absorb less water and degrade more slowly than PLGA containing a higher percentage of glycolic acid (Miller et al., 1977). PLGA degradation rate is also affected by the polymer molecular weight, the polydispersity index, and the nature of the end-chain (Makadia and Siegel, 2011).8
Synthesis
By adjusting the ratio of lactide to glycolide, several variants of PLGA can be obtained. The two widely used techniques to synthesize PLGA are:
1) Direct poly-condensation reaction above 120 degree Celsius to obtain low molecular weight PLGA. The process is however complex and is expensive to carry out. Moon and Takahashi introduced new melt and melt/solid poly-condensation process, without any azeotropical solvents, to overcome the limitations of direct poly-condensation method.
2) Ring-opening polymerization or poly-addition is used to achieve high molecular weight PLGA. The process incorporates the use of catalyst agents like 2-ethylhexanoate, tin (II) alkoxides, or aluminum isopropoxide and stannous octoate (SnOct2).9
Physico-Chemical Properties
The physiochemical properties of PLGA depends on several factors like molar ratio of lactide and glycolide, the storage temperature, the molecular weight, stereochemistry, characteristic of surrounding environment and the exposure time to water. This block polymer is amorphous or semi-crystalline in nature and can be fabricated into any size and shape. Also, it exhibits excellent tensile strength and modulus of elasticity. The polymer is soluble in various solvents like acetone and acetate. It undergoes hydrolysis when placed in water. The methyl side chains of PLA make them less soluble in water, hence PLGA with high ratio of PLA degrades more sluggishly. Degradation and drug-release rates are slower for lactic acid-rich PLGA polymers. The free carboxyl end-groups of PLGA can be used for chemical modifications to modulate its degradation rate or drug delivery properties. Hence, has been used to coat various biomolecules and also successfully in drug delivery. Several parameters like glass transition temperature, molecular weight and moisture content can change over time. It has been hypothesized that a high glycolic acid permits higher degradation rate. Another school of thought proposed was that a higher crystalline poly lactic acid (PLLA) also accelerates degradation. Park et al. commented that PLGA with a higher molecular weight and longer polymer chains exhibit slower degradation. Holy et al. showed that strong acidic or alkaline solution rapidly degrades the polymer. Also, several enzymes have been used to hasten PLGA degradation.
PLGA in Hydoxyapatite (HAP) Bone Grafts
Hydroxyapatite is considerably used in the medical world as it demonstrates both osteoconductive and osteoinductive properties. Even though it is considered the most influential material in the regeneration era, it brings certain shortcomings like- variation between bone degeneration rate and regeneration rate, ingrowth limit due to pore size, and lack of mechanical strength.10 This restrains its clinical application. Attempts are being made to overcome these obstructions by modifying the graft with organic and inorganic components. When PLGA particles are loaded in this graft, it leads to improvement in the mechanical strength in the initial phase. Gradually, the PLGA particles degrade to expose the macropores that forms interconnected pores. The strengthening of graft takes place by bony ingrowth and deposition of new bone should offset the weakening of graft due to polymer degradation.11 Different composition of this composite has been studied and published.
Andre et al., in an in vitro study compared PLGA scaffolds with PLGA/ hydroxyapatite composite. Scaffolds were initially characterized by Scanning electron microscopy (SEM) and then cytotoxicity and cell adhesion were checked on osteoblasts. Collagen synthesis was measured by Sirius Red colorimetric method. The authors concluded that the composite scaffold showed a better cellular response.12 An in vivo study by Kim et al. was conducted to fabricate and characterize PLGA/ βTCP (β Tricalcium Phosphate) by using two scaffold designs depending on deposition angulation. This graft was placed in a rabbit’s femoral defect and after 12 weeks, the samples were sent for histological examination. Their results demonstrated that the graft was taken up well at the site of defect.13
Bhuiyan et al. in their in vitro study developed nano haP-PLGA films on which human mesenchymal stem cells were seeded. They demonstrated that this system was a favourable environment for the growth of collagen, mineral deposition and expression of Alkaline Phosphatase (ALP) levels.14 Momir et al. conducted an in vivo study on 24 subjects presenting with chronic periodontitis with intrabony defects to evaluate and compare the efficacy of biphasic calcium phosphate-PLGA composite against beta tricalcium phosphate. Their results suggested that biphasic calcium phosphate-PLGA composite was a better choice for periodontal regeneration.15
On contrary, Marie et al. proposed that there was no difference between HA/PLGA and TCP when the amount of bone formation was evaluated. They conducted an in vitro study to evaluate the properties of both grafts by SEM, Transmission electron microscopy (TEM), X-ray diffraction (XRD) and Fourier-transform infrared spectroscopy (FTIR). Cytotoxic analysis was carried out on ovarian cells. The two grafts demonstrated good biocompatibility. Further an in vivo study was carried out in rat’s calvaria to assess regeneration. On intergroup comparison, the results obtained were however non-significant.16
Bone Morphogenic Protein (BMP)/PLGA Bone Grafts
BMP, a member of Tumor growth factor-beta (TGF-β) superfamily, is involved in several imperative vital processes occurring throughout the body. The signaling mechanism, morphogenesis, organogenesis, regulation and homeostasis are some of the very important functions of BMP. To exploit its regenerative potential, several materials have been added to BMP to formulate various combinations that have been used for scientific research.17,18
To cite, Haider et al. conducted an in vitro study where they formulated and grafted BMP-2 onto the surface of L-glutamic acid modified hydroxyapatite incorporated into the PLGA nanofiber matrix. This material was checked for the characterization using various spectroscopy and cytocompatibility tests. They observed that not only this material projected enhanced osteoblastic activity but also produced calcium at the defect site. Hence, the authors concluded that the graft could be used in various bone related disorders.19
Similarly, Zheng et al. used BMP-2 coupled with 2% nano-silver particles-PLGA composite grafts in infected femoral defects of rats and found that the sites healed in 12 weeks. No evidence of bacteria could be appreciated in the experimental site. Therefore, the authors concluded that, in addition to regenerative properties of this graft, a prolonged antibacterial effect was observed.20
Magnesium/PLGA Composite
Magnesium, the fourth most abundant mineral in our body, has osteopromotive actions with good biodegradability. When combined with other bone substitutes (bioglasses, bioceramics), Magnesium/ PLGA composite have demonstrated actions like osteogenesis and crystal growth.
In a research, Brown et al. prepared scaffolds of magnesium with PLGA in various ratios. The goal of the study was to check the mechanical and biological properties via characterization, cytocompatibility assay, degradation tests and micro-CT analysis. It was observed that the addition of magnesium increased the compressive strength. It was also seen that the amalgamated composite grafts had a porous structure which was suitably receptive for infiltration of osteoblasts. When this graft was placed in extraction socket, superior healing potential was appreciated. So, the authors concluded that the material could be used for socket and ridge preservation.21
Likewise, Yuan et al. conducted a study to evaluate the efficacy of PLGA microspheres with magnesium carbonate and magnesium oxide in different ratios (1:0, 3:1, 1:1, 1:3, 0:1). Several assays like cell viability, in vitro mineralization and characterization were carried out. All prepared grafts were non-cytotoxic and promoted osteogenic differentiation, cell migration and mineral deposition. The findings suggested that these grafts could be used in bone defects.22
Titanium/PLGA Composite
Titanium is a mineral that is widely distributed in earth’s crust. Titanium comes with the unique property of corrosion resistance and excellent biocompatibility. It has been used in various dental procedures like dental implants owing to its properties of bone augmentation and osseointegration. Titanium also has the ability to shape itself according to any bone defect for higher acceptability. Hence, researchers have always tried to modify this element to obtain the best results.
Shengjun et al., to modify the surface topography of implants designed Titanium oxide nanotubes impregnated with recombinant BMP2 (rhBMP2) (by vacuum freeze drying) and PLGA. The drug release rate was checked along with other investigations like cell adhesion, cell proliferation, immunofluorescence assay and western blot. This composite released BMP2 for four weeks. Also, the authors observed that there was an increase in pre-osteoblastic activity in the presence of this material. Moreover, the authors suggested that for better results, the thickness of PLGA should range between 50 nm and 250 nm.23
Smith et al. conducted an in vitro study where Titanium was coated with nano rough PLGA to improve the performance of Titanium. The same was evaluated for osteoblast cell density. They concluded that this material could be used in orthopedic implants as it resulted in enhanced osseointegration.24
PLGA/Bioactive Glass Composite
Another most commonly used commercially available bone graft material is the bioactive glass. It portrays different bioactivity at different compositions. Yang et al. conducted primarily an in vitro study to evaluate the potential of PLGA/bioactive glass composite on human osteosarcoma cells. The composite was characterized by SEM and its biocompatibility and biodegradability were checked on human osteosarcoma cell lines. Results suggested that this composite had a nano-fibrous three-dimensional structure with high porosity. He further conducted an in vivo study where the graft was implanted in rabbit’s ears. The results suggested that this composite raised the alkaline phosphatase level in the surrounding bone matrix with increased vascular activity.25
Kunwong et al. in an in vitro study checked the biocompatibility of this graft on human exfoliated deciduous teeth stem cells. The properties of the graft were checked by SEM, spectroscopy, pH analysis, etc. The authors concluded that the graft demonstrated an increase in pore size, improved hydrophilicity and improved mechanical properties with increased osteogenic activity.26
PLGA/Growth Factors
Growth factors mediate crosstalks between cellsproliferation, differentiation and mitogenesis. For the cell growth, development and survival, it is a crucial factor that cannot be neglected. When infused with bone grafts, it shows better healing and regeneration.27
In an in vivo study by Devi et al., a membrane was fabricated comprising of Insulin like growth factor (IGF), Vascular endothelial growth factor (VEGF), Tricalcium Phosphate (TCP), and PLGA. They concluded that the incorporation of growth factors in the membrane enhanced the capacity with better clinical output.21An in vitro study was conducted by Yoshida et al. where he designed a scaffold made of TCP, PLGA loaded with Fibroblast Growth factor (FGF-2). The above mentioned graft was characterized using TEM and X-ray diffraction. Then this was placed in rat’s cranial defects. The samples were sent for histological examination 10 and 35 days after surgery. Their results suggested that the graft had good mechanical strength with enriched bone augmentation properties.28
Duruel et al., performed an in vitro study where PLGA was combined with IGF-1, BMP-6, alginate and chitosan to form a scaffold. Characterization of micro-particles and cell culture assays were done to assess the bone response. Both alginate and PLGA showed controlled release of the growth factors, thereby indicating that in presence of growth factors both osteoblasts and cementoblasts exhibit an accelerated action.29
PLGA/Antimicrobials
Infection remains the major challenge in surgeries. To combat this, several trials of antimicrobials incorporated in bone grafts were carried out. Bulent et al. in their in vivo study compared the efficacy of two periodontal therapies, root planing (control group) against metronidazole loaded in PLGA membrane (test group). The membranes were used for guided tissue regeneration. After 60 days of periodontal surgery, histometric analysis was conducted. The results exhibited a better clinical outcome in the test group when histometric and clinical parameters like defect height, apical extension of junctional epithelium, new cementum height, new bone height and new gingival connective tissue height were taken into account.30 Yihui et al. conducted an in vitro and in vivo study on the periodontium of rats to evaluate the osteogenic effect of minocycline loaded PLGA membrane. The parameters were recorded by confocal microscopy, contact angle and SEM. The membrane was placed in rats where experimental periodontitis was induced. At 3 and 6 weeks after surgery, the samples were sent for bone analysis and immunohistochemistry. Their results demonstrated a sustained release of the drug (minocycline) and also the expression of osteoprotegerin was observed. The authors concluded that this material enhanced periodontal regeneration.31
Others
Kim et al. executed an innovative approach to PLGA by combining it with magnesium hydroxide and bone-extracellular matrix along with, bioactive poly-deoxyribonucleotide. The above scaffold was prepared by freeze drying method. It was characterized by SEM and thermal gravimetric analyser and was tested for cytotoxicity, wound healing properties, tube formation, Reverse transcriptase polymerase chain reaction (RT-PCR) and tartrate resistant acid phosphatase staining. The findings of the study suggests that apart from the osteogenic and angiogenic properties, the graft also suppressed the osteoclastic activity and pro-inflammatory cytokines. Hence the graft showed anti-inflammatory effect and provided ideal microenvironment for bone growth.32
Current Trends and Future Prospects Pure PLGA presents with low hydrophilic nature and reduced bioactivity, which is a drawback for its effective application. To combat this limitation, numerous manipulation approaches such as grafting bioactive groups onto the polymer surface or blending/ copolymerizing the polymer with other materials have been tried out to bestow the polymer with preferred properties. Surface modification of PLGA enables to attract desirable cells towards the interested area and create a suitable interface between the PLGA & surrounding tissues.33
Several, studies have been done to modify this PLGA and make them suitable bone graft substitute. Wan et al., in his study showed that synthesizing PLGA with oxygen plasma not only increased negative charge but also induced an etching effect that raised the hydrophicity of that polymer which in turn improved the cell adhesion properties.34 Qu et al., on the other hand, after the oxygen treatment, incubated the biomaterial in a modified simulated body fluid (SBF) solution which prompted the growth of apatite crystals.35 Yoon et al., fabricated PLGA and functionalized PLGA films scaffold by solvent coating and gas foaming methods. The above materials showed excellent biomimetic properties.36 Lee & his colleague synthesized barrier membrane incorporating PLGA and bone forming peptide. The product exhibited an elevated ALP level and enhanced calcium deposition.
Sterilization
Many methods were adopted to sterilize PLGA bone grafts over time like ethylene oxide (ETO), gamma sterilization and radio-frequency glow discharge (RFGD) plasma sterilization (low-temperature radio-frequency glow discharge plasma treatment). Studies have shown that by far, RFGD is the most efficient way to sterilize bone grafts. This method uses argon as a medium. ETO is a chemical disinfectant and cannot be used in in vivo sterilization of biomedical components due to its toxic residues. At the same time, the degradation rate was higher if PLGA was sterilized by gamma radiation.37
Limitations
1. The cost of production is high which tends to burden the patient with economic stress.
2. Different ratios are available for the product. It is yet to be discovered which one would be best for regeneration.
3. Also, some studies have quoted that it creates a local acidic environment that hampers the cell proliferation and repair activity.38
Conclusion
Despite the limitations, one cannot ignore the charm of these polymers. This article provides an insight into the relevance of PLGA in regeneration. Its noteworthy progress has made it a strategic material in bone tissue engineering. Owing to its versatility, configuration and flexibility, this material has found its applications in periodontology, dental surgery and in endodontic therapy. Further research should be carried out to make it the most ideal regenerative material.
Conflict of Interest
None
Supporting File
References
- Lü JM, Wang X, Marin-Muller C. Current advances in research and clinical applications of PLGAbased nanotechnology. Expert Rev Mol Diagn 2009;9(4):325-341.
- Roberts TT, Rosenbaum AJ. Bone grafts, bone substitutes and orthobiologics: the bridge between basic science and clinical advancements in fracture healing. Organogenesis 2012;8(4):114-24.
- Fukuba S, Okada M, Nohara K, Iwata T. Alloplastic bone substitutes for periodontal and bone regeneration in dentistry: Current status and prospects. Materials (Basel) 2021;14(5):1096.
- Makadia HK, Siegel SJ. Poly Lactic-co-Glycolic Acid (PLGA) as biodegradable controlled drug delivery carrier. Polymers 2011;3(3):1377-1397.
- Zhao D, Zhu T, Li J, Cui L, Zhang Z, Zhuang X, et al. Poly (lactic-co-glycolic acid)-based composite bone-substitute materials. Bioact Mater 2021;6(2):346-360.
- Hines DJ, Kaplan DL. Poly (lactic-co-glycolic) acid-controlled-release systems: experimental and modeling insights. Crit Rev Ther Drug Carrier Syst 2013;30(3):257-276.
- Blasi P. Poly (lactic acid)/poly(lactic-co-glycolic acid)-based microparticles: an overview. J Pharm Investig 2019;49:337-346.
- Lagreca E, Onesto V, Di Natale C, La Manna S, Netti PA, Vecchione R, et al. Recent advances in the formulation of PLGA microparticles for controlled drug delivery. Prog Biomater 2020;9(4):153-174.
- Silva ATCR, Cardoso BCO, Silva MESR, Freitas RFS, Sousa RG. Synthesis, characterization, and study of PLGA copolymer in vitro degradation. Journal of Biomaterials and Nanobiotechnology 2015;6(1):8-19.
- Jeong J, Kim JH, Shim JH, Hwang NS, Heo CY. Bioactive calcium phosphate materials and applications in bone regeneration. Biomater Res 2019;23(4):2-11.
- Simon Jr CG, Khatri CA, Wright SA, Wang FW. Preliminary report on the biocompatibility of a mouldable, resorbable, composite bone graft consisting of calcium phosphate cement and poly(lactide-co-glycolide) microspheres. J Orthop Res 2002;20(3):473-482.
- Dutra Messias A, Aragones A, Aparecida de Rezende Duek E. PLGA-hydroxyapatite composite scaffolds for osteoblastic-like cells. KEM 2008; 396(398):461-464.
- Kim J, McBride S, Tellis B, Alvarez-Urena P, Song YH, Dean DD, et al. Rapid-prototyped PLGA/β-TCP/hydroxyapatite nanocomposite scaffolds in a rabbit femoral defect model. Biofabrication 2012;4(2):1-11.
- Salles CM, Yoshimoto M, Allegrini M, Fancio S, Higa E, Suzuki O, et al. Physico/chemical characterization, in vitro, and in vivo evaluation of hydroxyapatite/PLGA composite and tricalcium phosphate particulate grafting materials. Titanium 2009;1(1):16-28.
- Bhuiyan DB, Middleton JC, Tannenbaum R, Wick TM. Bone regeneration from human mesenchymal stem cells on porous hydroxyapatite-PLGAcollagen bioactive polymer scaffolds. Biomed Mater Eng 2017;28(6):671-685.
- phosphate/poly-DL-lactide-coglycolide for periodontal intrabony defects filling. Vojnosanit Pregl 2015;72(8):689-95.
- James AW, LaChaud G, Shen J, Asatrian G, Nguyen V, Zhang X, et al. A review of the clinical side effects of bone morphogenetic protein-2. Tissue Eng Part B Rev 2016;22(4):284-97.
- Halloran D, Durbano HW, Nohe A. Bone morphogenetic protein-2 in development and bone homeostasis. J Dev Biol 2020;8(3):1-30.
- Haider A, Kim S, Man-Woo H, Inn-Kyu K. BMP-2 grafted nHA/PLGA hybrid nanofiber scaffold stimulates osteoblastic cells growth. BioMed Res Int 2015;2015(1):1-12.
- Zheng Z, Yin W, Zara JN, Li W, Kwak J, Mamidi R, et al. The use of BMP-2 coupled - NanosilverPLGA composite grafts to induce bone repair in grossly infected segmental defects. Biomaterials 2010;31(35):9293-9300.
- Brown A, Zaky S, Ray H Jr, Sfeir C. Porous magnesium/PLGA composite scaffolds for enhanced bone regeneration following tooth extraction. Acta Biomater 2015;11:543-53.
- Yuan Z, Wei P, Huang Y, Zhang W, Chen F, Zhang X, et al. Injectable PLGA microspheres with tunable magnesium ion release for promoting bone regeneration. Acta Biomater 2019;85:294-309.
- Sun S, Zhang Y, Zeng D, Zhang S, Zhang F, Yu W. PLGA film/Titanium nanotubues as a sustained growth factor releasing system for dental implants. J Mater Sci Mater Med 2018;29(9):141.
- Smith LJ, Swaim JS, Yao C, Haberstroh KM, Nauman EA, Webster TJ. Increased osteoblast cell density on nanostructured PLGA-coated nanostructured titanium for orthopedic applications. Int J Nanomedicine 2007;2(3):493-499.
- Yang L, Liu S, Fang W, Chen J, Chen Y. Poly (lactic-co-glycolic acid)-bioactive glass composites as nanoporous scaffolds for bone tissue engineering: In vitro and in vivo studies. Exp Ther Med 2019;18(6):4874-4880.
- Kunwong N, Tangjit N, Rattanapinyopituk K, Dechkunakorn S, Anuwongnukroh N, Arayapisit T, et al. Optimization of poly (lactic-co-glycolic acid)- bioactive glass composite scaffold for bone tissue engineering using stem cells from human exfoliated deciduous teeth. Arch Oral Biol 2021;123(5):1-10.
- Greenhalgh DG. The role of growth factors in wound healing. J Trauma 1996;41(1):159-167.
- Devi R, Dixit J. Clinical evaluation of insulin like growth factor-i and vascular endothelial growth factor with alloplastic bone graft material in the management of human two wall intra-osseous defects. J Clin Diagn Res 2016;10(9):41-46.
- Yoshida T, Miyaji H, Otani K, Inoue K, Nakane K, Nishimura H, et al. Bone augmentation using a highly porous PLGA/β-TCP scaffold containing fibroblast growth factor-2. J Periodontal Res 2015;50(2):265-273.
- Duruel T, Çakmak AS, Akman A, Nohutcu RM, Gümüşderelioğlu M. Sequential IGF-1 and BMP-6 releasing chitosan/alginate/PLGA hybrid scaffolds for periodontal regeneration. Int J Biol Macromol 2017;104(Pt A):232-241.
- Kurtiş B, Unsal B, Cetiner D, Gültekin E, Ozcan G, Celebi N, et al. Effect of polylactide/glycolide (PLGA) membranes loaded with metronidazole on periodontal regeneration following guided tissue regeneration in dogs. J Periodontol 2002;73(7): 694-700.
- Ma Y, Song J, Almassri H, Zhang D, Zhang T, Cheng Y, et al. Minocycline-loaded PLGA electrospun membrane prevents alveolar bone loss in experimental periodontitis. Drug Deliv 2020;27(1):151-160.
- Mano JF, Sousa RA, Boesel LF, Neves NM, Reis RL. Bioinert, biodegradable and injectable polymeric matrix composites for hard tissue replacement: State of the art and recent developments. Compos Sci Technol 2004;64(6):789-817.
- Wan Y, Qu X, Lu J, Zhu C, Wan L, Yang J, et al. Characterization of surface property of poly(lactide-co-glycolide) after oxygen plasma treatment. Biomaterials 2004;25(19):4777-83.
- Qu X, Cui W, Yang F, Min C, Shen H, Bei J, et al. The effect of oxygen plasma pretreatment and incubation in modified simulated body fluids on the formation of bone-like apatite on poly(lactide-coglycolide) (70/30). Biomaterials 2007;28(1):9-18.
- Yoon JJ, Song SH, Lee DS, Park TG. Immobilization of cell adhesive RGD peptide onto the surface of highly porous biodegradable polymer scaffolds fabricated by a gas foaming/salt leaching method. Biomaterials 2004;25(25):5613-5620.
- Kim DS, Lee JK, Jung JW, Baek SW, Kim JH, Heo Y, et al. Promotion of bone regeneration using bioinspired PLGA/MH/ECM scaffold combined with bioactive PDRN. Materials (Basel, Switzerland) 2020;14(15):41-49.
- Holy CE, Cheng C, Davies JE, Shoichet MS. Optimizing the sterilization of PLGA scaffolds for use in tissue engineering. Biomaterials 2001; 22(1):25-31.