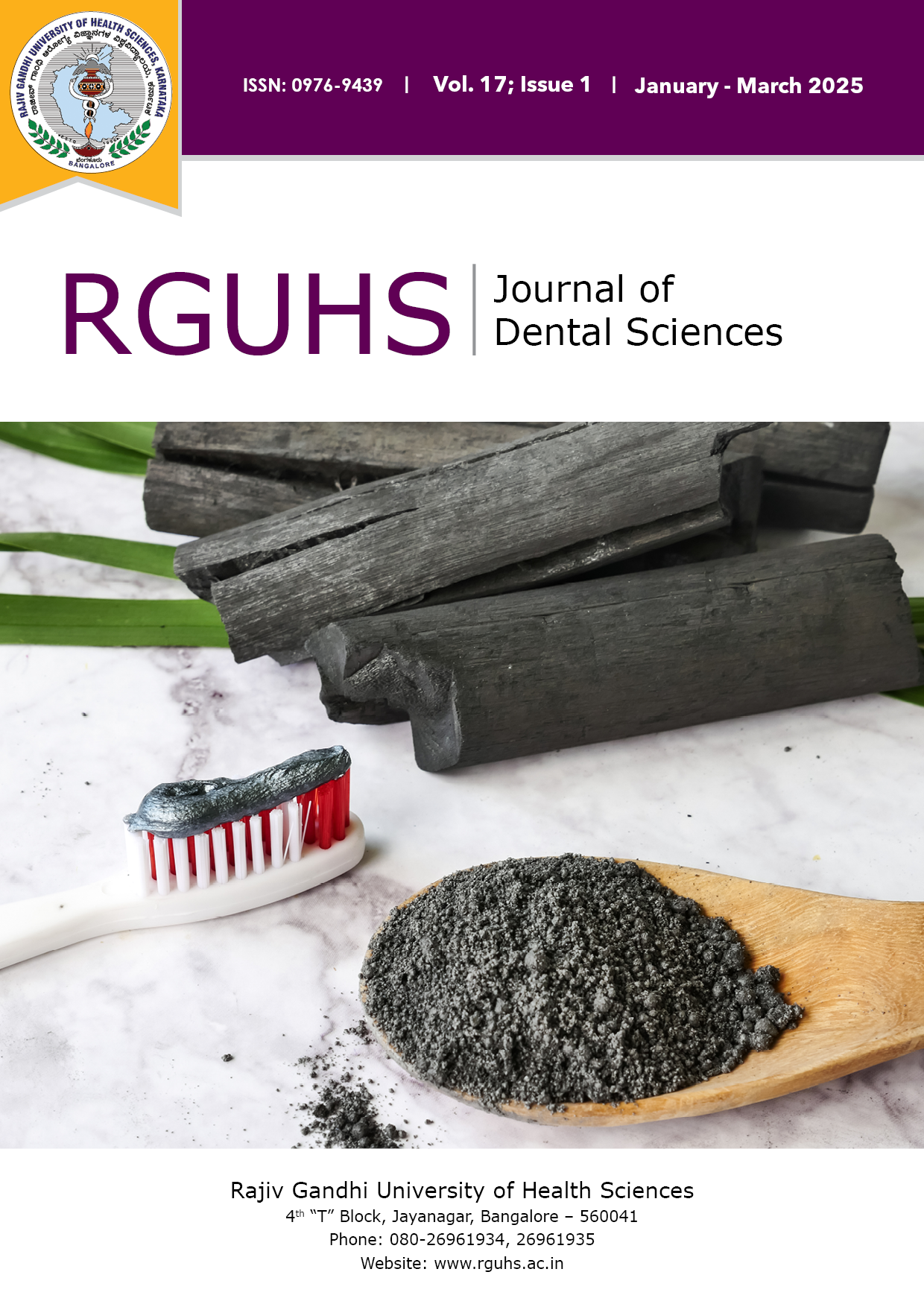
RGUHS Nat. J. Pub. Heal. Sci Vol No: 17 Issue No: 1 pISSN:
Dear Authors,
We invite you to watch this comprehensive video guide on the process of submitting your article online. This video will provide you with step-by-step instructions to ensure a smooth and successful submission.
Thank you for your attention and cooperation.
1Dr. Ancita Caroline Dsouza, Department of Prosthodontics, Crown & Bridge including Implantology, KVG Dental College & Hospital, Sullia, Karnataka, India.
2Department of Prosthodontics, Crown & Bridge including Implantology, KVG Dental College & Hospital, Sullia, Karnataka, India
3Department of Prosthodontics, Crown & Bridge including Implantology, KVG Dental College & Hospital, Sullia, Karnataka, India
*Corresponding Author:
Dr. Ancita Caroline Dsouza, Department of Prosthodontics, Crown & Bridge including Implantology, KVG Dental College & Hospital, Sullia, Karnataka, India., Email: ancitadsouza22@gmail.com
Abstract
Aim: The aim of this study was to evaluate and compare the fracture resistance and patterns of fracture in unreinforced, glass & aramid fiber mesh reinforced maxillary complete dentures under dynamic fatigue loading.
Methods: Forty-eight dentures were divided into three groups of 16 each. Unreinforced, Glass and Aramid fiber mesh-reinforced maxillary dentures were fabricated using heat polymerized polymethylmethacrylate. They were stored in artificial saliva for six months. Fatigue testing was performed using a chewing simulator with 100 N load for 2,40,000 cycles (corresponding to one year). Fracture resistance was evaluated using a Universal testing machine. Patterns of fracture were categorized as, complete, incomplete fracture (anterior & posterior fracture, anterior fracture, posterior fracture) and central deformation only (loaded area). Statistical analysis was conducted using descriptive statistics, ANOVA and Post-hoc Tukey's test.
Results: Aramid fibre mesh reinforced maxillary denture demonstrated the highest mean fracture resistance of 1561.82±198.49 N, followed by glass fibre mesh reinforced and unreinforced maxillary dentures with 1436.75±231.39 N & 1046.76±94.61 N, respectively. Unreinforced group showed complete fracture occurring in the midline, while the reinforced maxillary complete denture showed incomplete fracture.
Conclusion: Glass and aramid fiber mesh reinforcement significantly increased the fracture resistance of dentures. Although not statistically significant, aramid-reinforced dentures exhibited the highest fracture resistance than glass fiber. All intact fractures were noted with reinforced dentures, facilitating easy repair. These findings may improve the longevity and durability of dentures.
Keywords
Downloads
-
1FullTextPDF
Article
Introduction
Polymethyl methacrylate (PMMA) has been the standard denture base material since 1937 due to its superior strength, durability, dimensional stability, chemical stability, biocompatibility, repair ability, low water sorption, cost-effectiveness, ease of manipulation, simple processing technique, acceptable taste, and exceptional esthetics.1,2,3 Despite its popularity, this material lacks mechanical and physical requirements, particularly flexural strength, impact strength, fracture toughness and surface hardness, resulting in reduced prosthesis durability, patient dissatisfaction, polymerization shrinkage, midline fractures and tooth de-bonding.2-4
Metal frameworks were employed in clinical practice as reinforcement to complete denture, which enhanced their precision, volume stability and resistance to fracture. However, the use of these frameworks is limited due to their esthetic appeal, weight, complexity in fabrication and the potential for hypersensitivity caused by their alloy composition.5,6 The conventional approach involves metal plates or wires to reinforce the denture base acrylic resin. Metal reinforcement can slightly improve the transverse strength of polymers, but its impact on the fatigue resistance of dental appliances is minimal, leading to reoccurrence of denture base fractures.7,8
To improve the inherent mechanical characteristics of PMMA, efforts have been made to incorporate Butadiene styrene into the production of high-impact resin or by chemical modification giving maximum bulk to the material, by copolymerization, crosslinking and reinforcement with fibers like aluminum, sapphire whiskers, polycarbonates, graphite, silica, ceramic, mica, glass, carbon, Kevlar, aramid and polyethylene fibers or metal strengtheners in particulates, powder, whiskers and mesh form.9,10,11
Glass fibers have been explored due to their aesthetic appeal, flexibility, biocompatibility, quick fabrication, and lightweight compared to metal.2 They are also used as an alternative for metal framework to increase the repairability of failed dentures and due to their high elastic modulus, which allows bending without breaking and absorbs most stresses without deformation.12,13 They also possess improved mechanical characteristics such as transverse, tensile and impact strengths. Carbon fiber has a springy nature during handling and is less aesthetic due to undesirable black color.14 Aramids are organic fibers introduced for reinforcing the polymers because of their high mechanical properties such as chemical stability, thermal resistance, higher modulus of elasticity and tensile strength. They also demonstrate good mechanical stability, glass transition temperature, high melting point and limited solubility in different solutions.15 Being hydrophilic, they possess high surface energy, display good interfacial energy and have a superior compatibility with PMMA. Lee et al., revealed no indications of toxicity related to the use of polyaramid fibres.16
The clinical longevity of dentures is better predicted by dynamic loading than static loading. The purpose of the chewing simulators is to give information regarding a material's behaviour over extended periods of use. The physiological characteristics of human mastication, the direction and force of jaw movements are simulated to test the reinforced dentures before checking for fracture resistance in this dynamic fatigue loading machines.6,17
This study was an effort to evaluate and compare the fracture resistance of maxillary acrylic complete dentures with those reinforced with glass fibre mesh and aramid fibre mesh under dynamic fatigue loading followed by compressive loading.
Materials and Methods
Forty-eight acrylic maxillary dentures were fabricated which were divided into three groups of 16 specimens each.
GROUP A: Unreinforced maxillary dentures
GROUP B: Glass fiber mesh reinforced maxillary dentures
GROUP C: Aramid fiber mesh reinforced maxillary dentures
Heat polymerized PMMA (DPI, Mumbai) was utilized to fabricate maxillary denture on a standard edentulous cast according to the manufacturer’s guidelines incorporating a teeth set (Premadent M1 24). In order to standardize the specimens, an impression mould with elastomeric material (Photosil, DPI, Delhi) was made using this maxillary complete denture, and the same was then used to fabricate the remaining specimens (Figure 1). Identical teeth sets (Premadent M1 24) were used and each tooth was positioned in the indentation present in the elastomeric impression mold over which the molten modeling wax was poured. The stone cast was pressed into the mould, which included a relief hole to allow trapped air and excess wax to escape from the mould space. Once the mould had cooled, the duplicated wax denture model was retrieved.
Forty-eight such wax replicas were obtained using this method. The duplicated wax denture specimens were flasked and dewaxed using the conventional method. Heat polymerized polymethylmethacrylate was manipulated to dough stage and packed for the control group. The specimens were finished & polished and sixteen such dentures were obtained.
Glass fiber mesh (C-Glass, CT, Gujarat, India) and Aramid fiber mesh (Funique Composited Pvt Ltd.) were cut according to the size of the arch covering the entire palate, extending 3 mm from the crest of the ridge and 2 mm in front of the posterior border of the cast. The dough stage heat cure mix was equally placed between the two halves of the flask. The cellophane sheet was used for trial closure and subsequently removed. To create a pre-preg, glass fibre mesh was wetted with heat cure monomer and then sprinkled with polymer powder. Similar process was executed for Aramid fiber mesh. A well-adapted mesh was inserted in between the two halves of the flasks. The flasks were compressed at 1,000 N in the hydraulic press and processed. Dentures retrieved were polished (Figure 2 & 3).
Forty- eight maxillary complete denture samples were obtained. They were stored in artificial saliva (MP Sai Enterprises, Thane) for six months.
Dynamic fatigue loading was carried out using a chewing simulator (CS-4.8, SD Mechatronik). Loading at 100 N was applied by a custom-made stainless-steel T-bar shaped jig to the second premolar bilaterally at 1.6 Hz for 2,40,000 cycles (Figure 4). After fatigue testing, fracture status of each complete denture sample was noted.
The fracture resistance was assessed using a Universal testing machine (Zwick/Roell Z020). Dentures were loaded at a crosshead speed of 5 mm/min using a square-shaped rod after being positioned with the occlusal surface facing downwards. The load was applied to the tissue surface where the palatal midline of the denture crossed the line connecting the second premolars on both sides and a longitudinal line extending from the midline between the central incisors and the posterior border of the denture. Fracture resistance, expressed in Newtons, was the maximum force that withstood fracture. The test ended when the load fell 30% below the maximum load or when it fractured. The fracture resistance of all the groups was measured.
The fractured surfaces of the denture specimens broken into pieces were examined. The patterns of fracture were categorized as, complete fracture into two or multiple parts, incomplete fracture (anterior & posterior fracture, anterior fracture, posterior fracture), and central deformation only (loaded area). Other distinct features were also analyzed.
Statistical analysis was carried out using Statistical Package for Social Sciences (SPSS) version 27.00 [(IBM corp. Armonk, NY, USA released 2019)]. The mean fracture resistance and standard deviation were calculated for each group and compared.
Results
A total of 48 dentures were subjected to dynamic fatigue loading, thereafter evaluation of fracture resistance and patterns of fracture were analyzed.
A chewing simulator was used for fatigue testing in which a T-bar shaped jig applied 100 N of load on either side of the second premolar at 1.6 Hz for 2,40,000 cycles. Total cycles were intended to correspond to one year of ageing. There was no evidence of fracture among the 48 complete dentures after cyclic loading. When loading was applied, the unreinforced control group exhibited minor bending, but the reinforced complete dentures did not display any visible alterations throughout fatigue testing. Because the elastic moduli of both the fiber meshes were greater than the acrylic resin, the reinforced base group maintained its rigidity. Hence, it is clinically appreciated for the fact that the fiber mesh reinforced denture causes less injury to denture supporting tissues, as it deforms less during mastication. Therefore, the results of the dynamic fatigue loading imply that the fit of the denture to the supporting tissues deteriorated on wearing the dentures. But, the problem of failure or cracking might not arise if mastication is maintained at a typical chewing force for 10-14 months.
In the study, unreinforced maxillary dentures exhibited a mean fracture resistance of 1046.76±94.61 N. Glass fiber mesh reinforced maxillary denture showed an increased mean fracture resistance up to 1436.75±231.39 N. Similarly, Aramid fiber mesh reinforced maxillary denture demonstrated a highest mean fracture resistance of 1561.82N±198.49 N. Analysis of Variance (ANOVA) was conducted to determine the overall difference in fracture resistance among the groups (Table 1).
The results revealed that the fracture resistance varied significantly (P <0.001) among the three groups. Post-hoc Tukey’s test was performed to further analyze pairwise differences between the groups. The pairwise comparisons showed significant differences in fracture resistance between unreinforced maxillary dentures and both glass fiber (P <0.001) and aramid fiber reinforced dentures (P <0.001) (Table 2). The mean difference in fracture resistance between unreinforced and glass fiber reinforced dentures was ‑389.99 N, indicating a higher fracture resistance for the latter. Similarly, the mean difference between unreinforced and aramid fiber reinforced dentures was ‑515.06 N indicating an even higher fracture resistance with aramid fibers. Comparing the two types of reinforced dentures, there was no statistically significant difference between glass and aramid fiber reinforced dentures (P=0.145). The mean difference in fracture resistance between these two groups was 125.07 N, with slightly higher resistance observed in aramid fiber reinforced dentures, although not statistically significant.
Pattern of fracture in the unreinforced maxillary denture group occurred with central deformation at the loaded area, followed by cracks, finally resulting in a complete fracture (100%) occurring in the midline. All the dentures were fractured into several pieces of different sizes and patterns. Nevertheless, there was no complete fracture seen in any of the reinforced dentures. In the glass fiber mesh reinforced dentures (Group B), cracks initiated at the loaded area and the fracture line progressed further to only anterior region (18.75%), only posterior region (12.5%), and anterior & posterior region (43.75%). Few samples with glass fiber mesh reinforcement also showed fracture in the loaded region alone (25%), whereas in the Aramid fiber mesh reinforced dentures (Group C), cracks appeared in the loaded area and the fracture line progressed either to anterior (44%) or posterior (25%) regions causing a partial intact fracture. Central deformation (31%) at the loaded area was also observed. Aramid fiber mesh reinforced dentures did not exhibit a fracture extending from anterior to posterior region. Both the reinforced groups exhibited intact failures where the fibres remained unaffected, allowing the denture to maintain its shape. Delamination of mesh from the acrylic was also not observed.
Discussion
Reinforcement with fibers in polymethylmethacrylate relies on the concept that a soft and ductile polymer matrix transfers applied load to fibers via shear forces at the interface. The fibers act as the principal load-bearing elements with the matrix forming a continuous phase to surround and hold the fibers in place.
Glass fiber has been used as a reinforcement material in many fields due to its exceptional properties. There are two ways to increase the strength of glass fiber - unidirectional glass fiber or woven glass fiber mesh. Smith (1957) stated that adding isolated fibers to the dough or laminating with glass cloth are two ways for achieving glass reinforcement of PMMA. Aramid, also known as Kevlar, in comparison to carbon fiber, nylon and E-glass, possesses a higher elastic modulus and tensile strength. Due to its exceptional wettability, polyaramid fiber does not need treatment with a coupling agent.
According to Beyli MS et al., dentures absorb water and saliva. During the first three months of immersion, a linear dimensional change of approximately 0.3% in the flange-to-flange dimensions can be noted. This will have an impact on deformation under load, and long-term water sorption can reduce the fatigue resistance.18 Thus, the dentures were stored in artificial saliva for six months. Vallittu et al., stated that surface imperfections and water absorption reduced fatigue resistance.19
The resistance of polymers to crack initiation and pro-pagation can be measured using a fracture mechanics technique. The impact test is a widely used technique in the literature for estimating the fracture resistance.20 They are conducted at a very high speed, whereas the clinical failures of dentures are caused due to a slow fatigue phenomena. Hence, the fracture toughness testing may be more appropriate than the impact test.
Flexural fatigue caused by cyclic deformation from masticatory forces can result in midline fractures, due to microcrack propagation at points where the load is concentrated.12 According to Beyli MS et al., the most stressed area is the lingual region near the incisors and the incisal notch can elevate stress, contributing to midline fracture of maxillary dentures.18 Matthews and Wain'O reported that the polished surface on the palatal aspect, behind the anterior teeth, experiences the highest stress during function, making it a major contributing factor to midline fractures.21
In this study, cracks initiated at the loading point, and no fractures occurred at the incisal notch because the load was applied to the midpalatal area of the intaglio surface. However, if the load had been applied bilaterally on the posterior teeth, it would result in flexure of dentures and a different pattern of fracture. All reinforced groups displayed incomplete (intact) and partial fractures, either at the loaded area or in the anterior or posterior midline where the shape of the denture was unaffected and the fibers were unbroken, thus enabling easy repair.
In the present study, glass and aramid fiber reinforced dentures showed significantly increased fracture resistance compared to unreinforced dentures (P<0.001). This resonates with the previous research by Vallittu et al., who found that the addition of glass fibers to denture base resin increased its flexural strength, outperforming the unreinforced denture.7 Anusavice et al., reported a significant increase in fracture toughness & fatigue resistance of the resin material when glass fiber was utilized to reinforce the denture.22 Similarly, aramid fiber has been found to enhance the mechanical properties of denture base resin, as demonstrated by Raszewski et al., where aramid fiber reinforced acrylic resin showed increased flexural resistance with increase in the number of fiber bundles, a finding that was also observed in the present study.15 The study found no significant difference in fracture resistance between glass fiber and aramid fiber reinforced dentures, despite previous studies suggesting otherwise. In a study by Jacob et al., the flexural strength of PMMA reinforced with glass, aramid, and nylon fibers was determined.23 Glass fiber-reinforced specimens had the maximum flexural strength, followed by aramid and nylon. This difference can be attributed to the change in spatial orientation of the fibers. The study design and testing methodology could also be attributed to the variations observed.
It is important to consider the clinical effects of fiber reinforcement while fabricating dentures. Dentures that are reinforced with fibres may last longer and require fewer repairs or replacements. Thus, the denture wearers will experience an improvement in their quality of life with increased satisfaction. This also allows for stronger, more fracture resistant dentures that are thinner and lighter, enhancing wearer comfort and aesthetic appeal. This is particularly advantageous in patients with limited interarch space.
The challenge of managing the fibers was a concern. The fibers frayed when they were cut using scissors, making it a challenge to precisely incorporate them. The location of the reinforcement shifted during the compression of acrylic resin into the mould due to its movement. Careful handling of fibres during packing, as well as careful denture finishing and polishing, can help prevent this.
The strength of this study includes the usage of multifaceted, three dimensional structured maxillary dentures as a specimen because single maxillary denture fractures are among the most frequent prosthodontic failures.24 Bar specimens, commonly used in previous studies, do not accurately simulate the clinical stress distribution and force dissipation that occurs on a denture.
The study also focused on dynamic fatigue loading using a chewing simulator. This replicates the cyclic loading experienced by dentures during mastication. By subjecting the dentures to repeated loading cycles until fracture, the study captures the long-term durability of the reinforced dentures. In this study, a vertical loading of 100 N was applied. According to studies, this loading is comparable to the bilateral masticatory force used by patients wearing complete mandibular and maxillary dentures.18 Cracks or fractures did not occur in the dentures subjected to cyclic loading. This can be attributed to the low fatigue loads of 100 N or 2,40,000 cycles of loading which was not enough to result in fatigue failure, such as fracture of dentures. The fatigue testing of 2,40,000 cycles used was equivalent to using dentures for 10-12 months.
The study highlights the potential of materials to enhance mechanical properties. However, the limitations of the study need to be acknowledged. In vitro nature of the study limits the potential to which the real-life scenario of the denture usage can be replicated. This study was limited to the maxillary denture which may not completely represent the challenges encountered with mandibular dentures. The influence of the fracture resistance in mandibular reinforced dentures is different as temporomandibular joint is closely associated with the mandible and is the primary origin of the masticatory forces. The masticatory forces are fairly compensated for with the use of dynamic fatigue loading. Parameters like change in temperature, exposure to saliva and variations in occlusal forces have not been addressed. The duration of dynamic fatigue loading may not fully capture the long-term performance of reinforced dentures. T-bar applied forces bilaterally during the fatigue loading; however, the forces are different intraorally during mastication.
In the future, alternative denture reinforcement systems including a combination of fibers, different incorporation techniques can be studied. With the advancements in the field of rapid prototyping, precise incorporation of reinforcing materials can be closely monitored to provide dentures addressing individual patient requirements.
The valuable insights provided by the present study in enhancing the mechanical properties of denture base resins can be a stepping stone for increasing the durability, longevity, patient and operator experience. Longitudinal studies focusing on innovative use of various materials for reinforcement can potentially solve the longstanding limitations of the current denture base resin, ultimately improving the quality of life for denture wearers.
Conclusion
Within the limitations of this study, glass and aramid fiber reinforcements significantly increased the fracture resistance of maxillary acrylic complete dentures compared to unreinforced maxillary dentures. Although, not statistically significant, aramid fiber reinforced maxillary dentures provided slightly higher fracture resistance than glass fiber-reinforced maxillary dentures. No fractures were observed after 2,40,000 cycles fatigue testing corresponding to 10-14 months of clinical usage. All intact fractures were observed in glass and aramid fiber reinforced maxillary denture groups facilitating easy repair.
Conflicts of Interest
Nil
Supporting File
References
1. Peyton FA. History of resins in dentistry. Dent Clin North Am 1975;19(2):211-22.
2. Rana MH, Shaik S, Hameed MS, et al. Influence of dental glass fibers and orthopedic mesh on the failure loads of polymethyl methacrylate denture base resin. Polymers (Basel) 2021;13(16):2793.
3. Abdulrahim R, Yanikoğlu N. Evaluation of fracture resistance for autopolymerizing acrylic resin materials reinforced with glass fiber mesh, metal mesh and metal wire materials: An in vitro study. Open J Stomatol 2022;12(2):33-41.
4. Praveen B, Babaji HV, Prasanna BG, et al. Comparison of impact strength and fracture morphology of different heat cure denture acrylic resins: An in vitro study. J Int Oral Health 2014;6(5):12-6.
5. Geurtsen W. Biocompatibility of dental casting alloys. Crit Rev Oral Biol Med 2002;13(1):71-84.
6. Im SM, Huh YH, Cho LR, et al. Comparison of the fracture resistances of glass fiber mesh- and metal mesh-reinforced maxillary complete denture under dynamic fatigue loading. J Adv Prosthodont 2017;9(1):22-30.
7. Vallittu PK. Flexural properties of acrylic resin polymers reinforced with unidirectional and woven glass fibers. J Prosthet Dent 1999;81(3):318-26.
8. Vallittu PK. A review of methods used to reinforce polymethyl methacrylate resin. J Prosthodont 1995;4(3):183-7.
9. Mansour MM, Wagner WC, Chu TM. Effect of mica reinforcement on the flexural strength and microhardness of polymethyl methacrylate denture resin. J Prosthodont 2013;22(3):179-83.
10. Kumar GV, Nigam A, Naeem A, et al. Reinforcing heat-cured poly-methyl-methacrylate resins using fibers of glass, polyaramid, and nylon: An in vitro study. J Contemp Dent Pract 2016;17(11):948-952.
11. Anne G, Mukarla NP, Manne P, et al. Comparative evaluation of flexural strength of conventional and reinforced heat cure acrylic resins: An in vitro study. J Dent Res Rev 2017;4(1):9-12.
12. Jayasree Komala DP, Bheri S. To evaluate the fracture resistance of maxillary complete dentures reinforced with full and partial glass fibre mesh: an in vitro study. Int J Med Health Res 2018;4(8):181-7.
13. Murthy HB, Shaik S, Sachdeva H, et al. Effect of reinforcement using stainless steel mesh, glass fibers, and polyethylene on the impact strength of heat cure denture base resin - An in vitro study. J Int Oral Health 2015;7(6):71-9.
14. Kattimani PT, Shah T, Pareek V, et al. A comparative study of palatal shapes and flexural properties of edentulous maxillary conventional heat polymerised acrylic resin denture bases with glass fibre/metal mesh reinforced bases. Journal of Dental and Medical Sciences 2019;18:51-70.
15. Raszewski Z, Nowakowska D. Mechanical properties of poured aramid fiber reinforced acrylic resin depending on fiber quantity, fiber position and different wetting agents. Dental and Medical Problems 2011;48(3):380-7.
16. Lee KP, Kelly DP, Kennedy GL Jr. Pulmonary response to inhaled Kevlar aramid synthetic fibers in rats. Toxicol Appl Pharmacol 1983;71(2):242-53.
17. Soriano-Valero S, Román-Rodriguez JL, Agustín-Panadero R, et al. Systematic review of chewing simulators: Reality and reproducibility of in vitro studies. J Clin Exp Dent 2020;12(12):e1189-e1195.
18. Beyli MS, von Fraunhofer JA. An analysis of causes of fracture of acrylic resin dentures. J Prosthet Dent 1981;46(3):238-41.
19. Vallittu PK. Comparison of the in vitro fatigue resistance of an acrylic resin removable partial denture reinforced with continuous glass fibers or metal wires. J Prosthodont 1996;5(2):115-21.
20. Zappini G, Kammann A, Wachter W. Comparison of fracture tests of denture base materials. J Prosthet Dent 2003;90(6):578-85.
21. Matthews E, Wain EF. Stresses in denture bases. Br Dent J 1956;100:167-71.
22. Anusavice KJ, Shen C, Rawls HR. Phillips' science of dental materials-e-book. Elsevier Health Sciences; 2012.
23. John J, Gangadhar SA, Shah I. Flexural strength of heat-polymerized polymethyl methacrylate denture resin reinforced with glass, aramid, or nylon fibers. J Prosthet Dent 2001;86(4):424-7.
24. Farmer JB. Preventive prosthodontics maxillary denture fracture. J Prosthet Dent 1983;50(2):172-5.