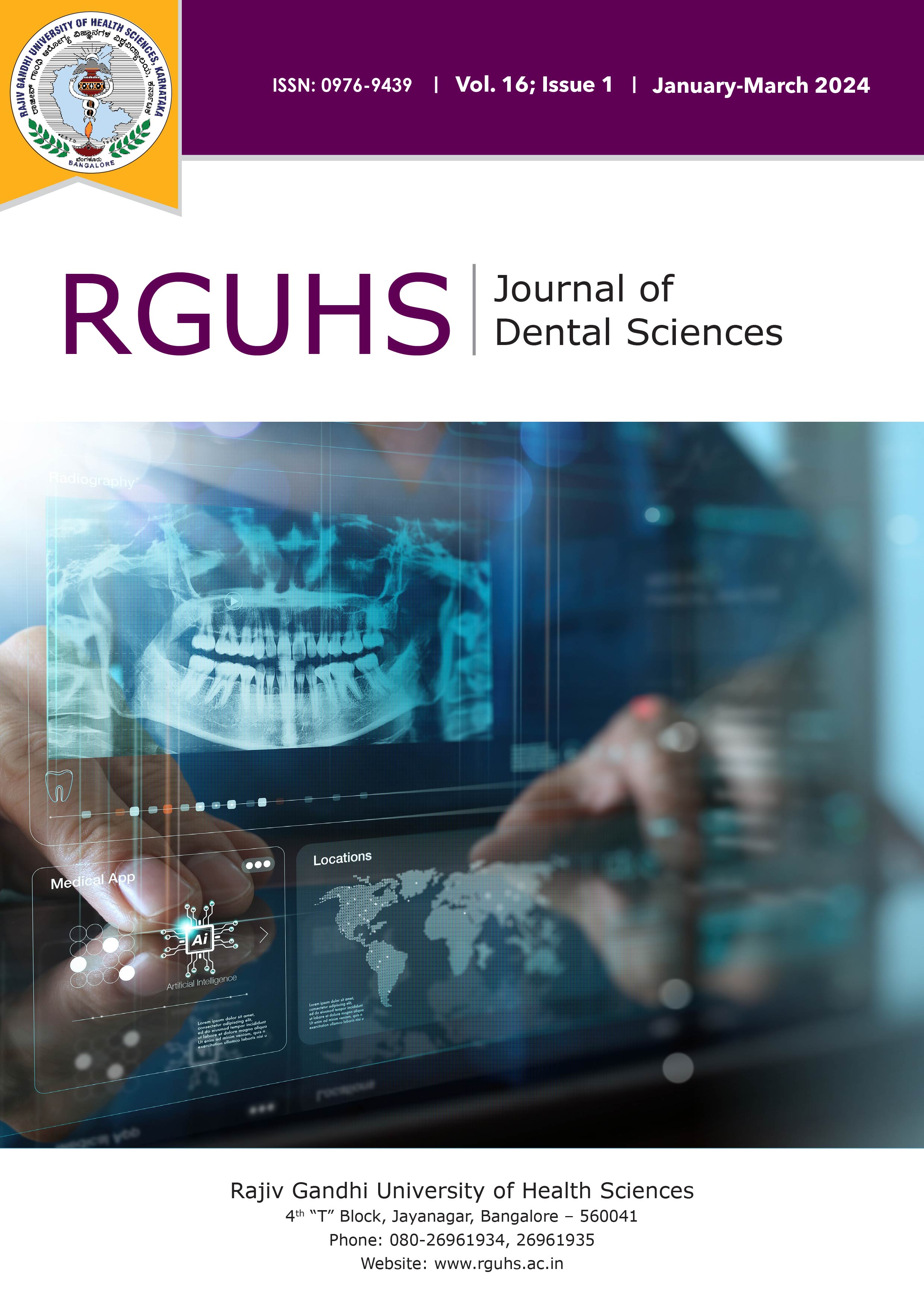
RGUHS Nat. J. Pub. Heal. Sci Vol No: 16 Issue No: 1 eISSN: pISSN:
P Roshan Kumar1*, N Kalavathy2 , Mitha M Shetty3 , Archana K Sanketh4 , Rutuja Tidke5
1 Reader, Department of Prosthodontics, D. A. Pandu Memorial R.V. Dental College, Bangalore.
2 Professor and Head, Department of Prosthodontics, D. A. Pandu Memorial R.V. Dental College, Bangalore.
3 Reader, Department of Prosthodontics, D. A. Pandu Memorial R.V. Dental College, Bangalore.
4 Reader, Department of Prosthodontics, D. A. Pandu Memorial R.V. Dental College, Bangalore.
5 Postgraduate student, Department of Prosthodontics, D. A. Pandu Memorial R.V. Dental College, Bangalore.
*Corresponding author:
Dr. P Roshan Kumar, Reader, Department of Prosthodontics, D. A. Pandu Memorial R.V. Dental College, Bangalore. Affiliated to Rajiv Gandhi University of Health Sciences, Karnataka- 560078, India. E-mail: dr_roshankumar_28@yahoo.co.in
Received date: May 30, 2021; Accepted date: June 28, 2021; Published date: June 30, 2021

Abstract
Three dimensional (3D) printing is the most widely used technology in reconstructive surgery to fabricate complex maxillofacial prosthesis. 3D Bioprinting, a combination of 3D printing and tissue engineering is a rapidly expanding technology in the field of regenerative medicine for autograft production. It is an additive manufacturing process in which there is computer-aided deposition of living cells with extracellular matrix (ECM) components with a biomaterial in particular combinations, for the fabrication of 3D biologically active tissue. In this review, we introduced the techniques, principles, limitations and future prospects of 3D bioprinting, a method that can open up new avenues in reconstructive surgery, by solving the problem of organ shortage and decreasing the donor site morbidity.
Keywords
Downloads
-
1FullTextPDF
Article
Introduction
Maxillofacial rehabilitation to re-establish aesthetic form and function has always been challenging to the maxillofacial prosthodontist. These deformities may negatively affect patient’s physical and psychological health, which can lead to serious psychiatric, familial, and social problems. These deformities can be congenital or acquired by pathologies such as necrotizing diseases, onco surgeries or trauma.1 Reconstructive surgeries are usually applied for the management of these defects. However, many a times prosthetic rehabilitation is still needed to restore the defect.1,2
Three dimensional (3D) printing, an additive manufacturing technology is currently widely employed to enhance the aesthetics of maxillofacial prosthesis with precise 3D construction.3 It uses computer aided design software to design complex facial geometries followed by layer-by-layer deposition of material to fabricate 3D objects.4 Not only can it fabricate complex craniofacial analogues, but also templates for bone grafts, osteotomy guides, intraoperative occlusal splints, which enhances efficiency and simplifies the surgery.5 But fabrication of indistinguishable maxillofacial prosthesis still remains a challenge.
Based on the principle of additive manufacturing, biomaterials, bioactive factors and even cells which are precisely positioned and with spatial control can be 3D printed to reconstruct human tissues and organs, that can mimic their native counterparts in terms of both structures and function called as 3D bioprinting.4 It is the combination of 3D printing and tissue engineering. Tissue engineering is a branch of regenerative medicine which aims to use patient’s own cells to create an autologous graft.6 Robert Langer and Joseph Vacanti defined tissue engineering as, ‘‘an interdisciplinary field that applies the principles of engineering and life sciences towards the development of biological substitutes that restore, maintain, or improve tissue function or a whole organ.’’7 The intrigue of 3D bioprinting could change the face of reconstructive surgery and prosthesis, with improved precision and suppressing the need for donor site or immunosuppressive treatments.7
3D bioprinting
Murphy and Atala described 3D bioprinting as, ‘‘layerby-layer precise positioning of biological materials, biochemicals and living cells, with spatial control of the placement of functional components (extracellular matrix, cells and pre-organized microvessels) to fabricate 3D structures.’’7
Method of bioprinting
The process of 3D bioprinting involves three stages – pre-processing, processing and post- processing7
1. Pre-processing – CAD tools are utilized for designing of mesh and to control the layer by layer deposition (microarchitecture) and overall shape of the model (macroarchitecture).
2. Processing – The bioink is used to print the model with suitable bioprinter in controlled parameters.
3. Post-processing – The printed model is then kept inside an incubator under specific conditions for maturation in which the model gets external growth factors and daily culture medium supply.
Bioink
A bioink is an integration of either differentiated cells or stem cells and fluidic biomaterial.7 It resembles the extracellular matrix containing cells, which when deposited precisely polymerizes or cross-links to form the scaffold. Previously, a single bioink deposition was possible but with the advancement of the field, multicomponent bioinks deposition in high accuracy is achieved to mimic the complex architecture of human tissues.8
The selection of the bioink relies on the particular application, the type of cells and the bioprinter to be used.9
Ideal requirements
(i) Should be capable of accurate, rapid and controlled layered deposition to form 3D structure.10
(ii) Biocompatibility – the material should have no harmful effects on host tissues with positive interactions with the host tissue.4
(iii) Should have adequate mechanical strength and robustness to resist external stress and retain the initial shape.4
(iv) It should have adjustable gelation and stabilization properties for different bioprinters.9
(v) Should be suitable for chemical modifications for tissue-specific needs and should be stable during sterilization.9
(vi) Should be capable of large-scale production with less variations within two product properties fabricated at different time intervals.9
(vii)Biodegradability – the material should gradually degrade in the body with the degradation rate matching the proliferation rate of new cells with non-toxic excrete.11
Types of bioinks9
1) Natural biomaterial based bioinks
2) Synthetic biomaterial based bioinks
3) Cell aggregate/pellet-based bioinks
4) Commercial bioinks – Dermamatrix, Novogel
5) Composite bioinks/bioinks with bioactive molecules - Magnetic iron oxide particles, blood plasma etc.
Cells
Autologous or primary cell types if used can mimic their in vivo counterparts, but are difficult to isolate and culture in vitro due to limited lifespan.12,13 Therefore, stem cells are preferred as they have the properties of self-renewal, pluripotency/multipotency and longevity representing an unlimited cell source for 3D bioprinting.12,14
Embryonic stem cells (ESCs) and induced pluripotent stem cells (iPSCs) are capable of differentiating into any cell type and are successfully used for the fabrication of tissue constructs. However, the risk of tumorigenicity and ethical issues is also a concern.15
Adult stem cells (ASCs) are undifferentiated stem cells derived from bone marrow, adipose tissues, and liver and are capable of self-renewal. Mesenchymal stem cells (MSCs) are one type of ASC that are believed to be less tumorigenic and are used extensively for 3D bioprinting.3
Solid tissues
(i) Cartilage and bone - Adipose-derived stem/stromal cells (ADSCs),16 mesenchymal stem cells (MSCs)17
(ii) Skin - Amniotic fluid-derived stem cells (AFSCs) and MSCs18
(iii) Muscles - Muscle-derived stem cells (MDSCs),19 MSCs, myoblasts20
Hollow tissues
(i) Vasculature - Human umbilical vein endothelial cells (HUVECs), MSCs
Others
(i) Cardiac tissues - Embryonic stem cells (ESCs),19 IPSC derived cardiomyocytes22, MSCs, Myoblasts
(ii) Neural tissues - Neural stem cells (NSCs),23 ESCs, MSCs, Glioma stem cells
Polymers
One of the most common materials used as bioink are polymers.
Advantages24
1. Low cost
2. Biocompatibility
3. Degradation
4. Secure processing
5. Ability to change their form
Natural polymers
1. Collagen: Most attractive and has been extensively employed in tissue engineering. It is the main protein component of musculoskeletal tissue and forms the extracellular matrix (ECM) of most tissues. Collagen is a triple-helical protein which provides superior microenvironments for cell growth, adhesion, and function. Generally, type I collagen is used.
2. Fibrin: Fibrin is involved in blood clotting and wound healing. It is used as scaffolding materials for vascular grafts. Fibrin gels have many favourable features like differentiation, biodegradability, promoting cell growth, angiogenesis, biocompatibility, and tissue regeneration, but is expensive.
3. Silk: Silk fibers produced by Bombyx mori (B. mori) are commonly used in tissue engineering. It has advantages like high biocompatibility, perfect mechanical stability, luster, non-toxicity, and low bacterial adherence. They have few disadvantages such as the need to mix with another polymer and need to optimize rheology of the bioink.
4. Chitosan: It is a sugar obtained from the hard outer skeleton of shellfish. It has advantages of its biocompatibility, antibacterial properties, biodegradability, and low price but disadvantages like weak mechanical integrity and rapid dissociation under certain conditions.
5. Alginate: It is a natural polysaccharide with properties like biocompatibility, low price, different choices of crosslinking, compatibility with various methods of printing and easy forming.
6. Gelatin: Gelatin is a natural protein that has an amphoteric behavior due to alkaline and acidic amino acid functional groups. Gelatin is noncytotoxic, water-soluble, promotes cell adhesion, biocompatible, has biodegradable properties, and low immunogenicity.
7. Hyaluronic acid: HA is a high molecular weight polysaccharide, and is the main component of the ECM. It has excellent properties that include biodegradability, bioresorbability, biocompatibility, and they are non-adhesive, non-thrombogenic and non-immunogenic.
Synthetic polymers25
1. Polycaprolactone: a polyester-based material with advantages of relatively low melting point, high stability, and long-term degradation, but it cannot encapsulate cells.
2. Polyethylene glycol: widely used in tissue engineering. It is a hydrophilic polymer with desirable properties of biocompatibility, nonimmunogenicity, and protein rejection properties.
3. Pluronic F-127: a water-soluble and thermoresponsive material with very weak mechanical integrity and provides poor cell support.
4. Polyvinyl alcohol: a biodegradable, biocompatible, thermostable, and water-soluble synthetic polymer, but with poor cell affinity which requires physical modification.
5. Polylactic acid and Poly Lactic-co-Glycolic acid: polyester-based polymers which are biodegradable, biocompatible but hydrophobic.
Bioprinters
1. Inkjet-Based Bioprinter24
It is one of the oldest and still widely used26 non-contact reprographic strategy based on the deposition of bioink drops. Continuous-inkjet bioprinting, electro-hydrodynamic jet bioprinting, and drop-on demand inkjet bioprinting are the three types of inkjet bioprinting, among which the drop on demand is most commonly employed.3 (Figure 1)
Advantages – Low viscosity biomaterials, faster speed,27 low cost, high resolution.3
Disadvantages – Poor vertical printing ability, no continuous flow.3
Types of drop on bioprinter
I. Piezoelectric bioprinters - generates acoustic waves within the bioink chamber using a piezoelectric actuator that ejects droplets through the printer nozzle.
II. The thermal method - contains a fluid chamber and single or multiple nozzles. Pressure pulses are formed by the heat generated within the bioink chamber, ejects a picoliter volume of droplets from the nozzle.
III. Electrostatic bioprinters - droplets are generated by voltage pulses, which are applied between a pressure plate and an electrode.
2. Laser-Based Bioprinting
Laser-based bioprinting, also known as laserinduced forward transfer bioprinting (LIFT bioprinting) was given by Bohandy et al. nearly 30 years back.28 A laser beam pulsating at controlled rates prints cells onto a receiving substrate for tissue construct fabrication. It consists of a laser beam, a focusing system, a laser absorbing ribbon, a receiving substrate, and a bioink.29 (Figure 2)
Advantages - High resolution, deposition of biomaterials both in solid or liquid forms.3
Disadvantages – Expensive, damage to cells, less cell viability.3
3. Extrusion-Based Bioprinting
It is also known as direct writing. Extrusion-based printing methods are of three types - pneumatic, piston-driven, and screw-driven dispensing.30 In pneumatic dispensing, air pressure provides the required driving force, while in piston and screw-driven dispensing, vertical and rotational mechanical forces initiate printing respectively, and it is extruded continuously as a strand.3 Here, a bioink is deposited on a substrate placed below the dispenser.
Advantages – Simple to use, various biomaterials can be printed, dense cells can be printed.3
Disadvantages – Slow,27 only suitable with viscous bioinks.3
4. Stereolithography (SL)
It uses a precisely controlled laser (commonly UV light) and a directed micromirrors array to project the light beam onto the surface of the light sensitive polymer. Because of the disadvantages of UV light such as carcinogenesis and its effects on DNA cells, visible light-based stereolithography is rapidly developing approach in bioprinting field. (Figure 4)
Advantages - Nozzle-free technique, less printing time, high accuracy and cell viability.3
Disadvantages – Toxicity to cells, cannot print multiple cells.3
5. Bioplotting
Bioplotting involves a syringe to extrude either tubes or spheroids of materials. These usually have several syringes and can employ multiple cell types and fabrication conditions resulting in the construction of multiple tissue types in the final construct, which is ideal for producing bioengineered soft tissues. Curing is done by either a chemical reaction or UV radiation of the tissue layers which are arranged on top of each other.
1. Fused-Deposition Modeling (FDM)
FDM is the oldest 3D additive manufacturing technology. It deposits a melted thermoplastic material in thin layers that provides support for hydrogel-based bioinks.
Limitations
1. There is a lack of a uniformity for choice of bioink, type of printer, parameters like temperature, oxygen, speed and maturation procedure.7
2. Tissue vascularization – till now proper tissue vascularization has not been achieved with any technique.7
3. The degree of similarity between 3D printed structure and present complex human structure is unclear.29
4. Inability to fabricate completely biocompatible bioink which will form multifunctional structures with appropriate mechanical strength with proper cellular viability.29
Future Prospects
1. Printing of specific functional tissues in vitro will help to check in vitro drug tolerance.7
2. Drug efficacy can be accurately checked on in vitro pathologic models.7
3. Printing functional models with defects would be of great help for teaching surgical and prosthetic management, simulating real life situation.7
4. Most of all, reconstructive surgery could be done with a small biopsy and bioprinting, rather than large grafts decreasing donor site morbidity.7
5. Autologous organs can be printed without waiting for donors.7
Discussion
In this article, we briefly reviewed on 3D bioprinting methods, bioinks which are currently used, the bioprinters and their uses. Meanwhile, future prospects as well as current limitations of 3D bioprinting were discussed. 3D bioprinting is a promising strategy to fabricate 3D tissues and organs in vitro using living cells and biomaterials.
Human organs consists of different types of cells, their matrices and their complex arrangement in an organ. At present, none of the existing bioprinters are capable of manufacturing total organ or tissues due to limitations of vascularity, printing speed, resolution and compatibility of biomaterials and physiological environment. Bioinks are crucial components of 3D bioprinting process, but their mechanical, rheological and biological properties are not up to the mark to satisfactorily meet bioprinting requirements. Also, properties of different types of biomaterials (eg. hydrogels) and polymers (eg. Alginate, gelatin) which are used to make bioinks needs improvisation.
Extensive research is being carried out in the field of 3D bioprinting over the past decade, which promises better future in tissue engineering. Challenges like vascularization, manufacturing issues and tissue survival can be overcome by further expansion of knowledge on multimaterial bioinks and their compatibility with physiological environment, flow properties and more precise and accurate bioprinting methods. Also, development of newer strategies which will evaluate and standardize bioinks and bioprinting process are necessary. In addition to this, development of new computational model for analysing bioink behaviors is also an important field of research. Development of this multidisciplinary approach might provide engineered tissues and organs to human recipients in the near future.
Conclusion
Even though 3D bioprinting is in its infancy stage, extensive research in the field of biotechnology, material science and nanotechnology is being carried out. Huge advancements in the fabrication of 3D printed organs can be predicted in the near future, once shortcomings of bioinks and biomanufacturing gets addressed. It can be predicted that bioprinting products will eventually become available in the market allowing reconstruction of large maxillofacial defects in situ, which will enhance the quality of life to a greater extent.
Conflict of Interest
None.
Supporting Files
References
- de Caxias FP, Dos Santos DM, Bannwart LC, de Moraes Melo Neto CL, Goiato MC. Classification, history, and future prospects of maxillofacial prosthesis. International journal of dentistry. 2019 Jul 18;2019.
- Naveau A, Smirani R, Rémy M, Pomar P, Destruhaut F. Cybergology and bioprinting: The biotechnological future of maxillofacial rehabilitation. International Journal of Maxillofacial Prosthetics. 2019;1:20-6.
- Derakhshanfar S, Mbeleck R, Xu K, Zhang X, Zhong W, Xing M. 3D bioprinting for biomedical devices and tissue engineering: A review of recent trends and advances. Bioactive materials. 2018;3(2):144- 56.
- Mao H, Yang L, Zhu H, Wu L, Ji P, Yang J, Gu Z. Recent advances and challenges in materials for 3D bioprinting. Progress in Natural Science: Materials International. 2020 Oct 28.
- Keyhan SO, Ghanean S, Navabazam A, Khojasteh A, Iranaq MH. Three-dimensional printing: a novel technology for use in oral and maxillofacial operations. In A Textbook of Advanced Oral and Maxillofacial Surgery Volume 3 2016 Aug 31. IntechOpen.
- Howard D, Buttery LD, Shakesheff KM, Roberts SJ. Tissue engineering: strategies, stem cells and scaffolds. Journal of anatomy. 2008;213(1):66-72.
- Sigaux N, Pourchet L, Breton P, Brosset S, Louvrier A, Marquette CA. 3D Bioprinting: principles, fantasies and prospects. Journal of stomatology, oral and maxillofacial surgery. 2019;120(2):128-32.
- Salah M, Tayebi L, Moharamzadeh K, Naini FB. Three-dimensional bio-printing and bone tissue engineering: technical innovations and potential applications in maxillofacial reconstructive surgery. Maxillofacial plastic and reconstructive surgery. 2020;42:1-9.
- Gungor-Ozkerim PS, Inci I, Zhang YS, Khademhosseini A, Dokmeci MR. Bioinks for 3D bioprinting: an overview. Biomaterials science. 2018;6(5):915-46.
- Jain S, Yassin MA, Fuoco T, Liu H, Mohamed-Ahmed S, Mustafa K, Finne-Wistrand A. Engineering 3D degradable, pliable scaffolds toward adipose tissue regeneration; optimized printability, simulations and surface modification. Journal of tissue engineering. 2020;11:2041731420954316.
- Nair LS, Laurencin CT. Biodegradable polymers as biomaterials. Progress in polymer science. 2007;32(8-9):762-98.
- Ong CS, Yesantharao P, Huang CY, Mattson G, Boktor J, Fukunishi T, Zhang H, Hibino N. 3D bioprinting using stem cells. Pediatric research. 2018;83(1):223-31.
- Zhang B, Gao L, Ma L, Luo Y, Yang H, Cui Z. 3D Bioprinting: A novel avenue for manufacturing tissues and organs. Engineering. 2019;5(4):777-94.
- Zhang YS, Yue K, Aleman J, MollazadehMoghaddam K, Bakht SM, Yang J, Jia W, Dell’Erba V, Assawes P, Shin SR, Dokmeci MR. 3D bioprinting for tissue and organ fabrication. Annals of biomedical engineering. 2017;45(1):148-63.
- Knoepfler PS. Deconstructing stem cell tumorigenicity: a roadmap to safe regenerative medicine. Stem cells. 2009;27(5):1050-6.
- Williams SK, Touroo JS, Church KH, Hoying JB. Encapsulation of adipose stromal vascular fraction cells in alginate hydrogel spheroids using a direct-write three-dimensional printing system. BioResearch Open Access. 2013;2(6):448-54.
- Chocholata P, Kulda V, Babuska V. Fabrication of scaffolds for bone-tissue regeneration. Materials. 2019;12(4):568.
- Skardal A, Mack D, Kapetanovic E, Atala A, Jackson JD, Yoo J, Soker S. Bioprinted amniotic fluid-derived stem cells accelerate healing of large skin wounds. Stem cells translational medicine. 2012;1(11):792-802.
- Phillippi JA, Miller E, Weiss L, Huard J, Waggoner A, Campbell P. Microenvironments engineered by inkjet bioprinting spatially direct adult stem cells toward muscle- and bone-like subpopulations. Stem cells. 2008;26(1):127-34.
- Yu H, Tay CY, Pal M, Leong WS, Li H, Li H, Wen F, Leong DT, Tan LP. A Bio-inspired Platform to Modulate Myogenic Differentiation of Human Mesenchymal Stem Cells Through Focal Adhesion Regulation. Advanced healthcare materials. 2013; 2(3):442-9.
- Bauwens CL, Peerani R, Niebruegge S, Woodhouse KA, Kumacheva E, Husain M, Zandstra PW. Control of human embryonic stem cell colony and aggregate size heterogeneity influences differentiation trajectories. Stem cells. 2008;26(9):2300-10.
- Ong CS, Fukunishi T, Nashed A, Blazeski A, Zhang H, Hardy S, DiSilvestre D, Vricella L, Conte J, Tung L, Tomaselli G. Creation of cardiac tissue exhibiting mechanical integration of spheroids using 3D bioprinting. Journal of visualized experiments: JoVE. 2017(125).
- Hsieh FY, Hsu SH. 3D bioprinting: a new insight into the therapeutic strategy of neural tissue regeneration. Organogenesis. 2015;11(4):153-8.
- Mobaraki M, Ghaffari M, Yazdanpanah A, Luo Y, Mills DK. Bioinks and bioprinting: A focused review. Bioprinting. 2020;18:e00080.
- Yu J, Park SA, Kim WD, Ha T, Xin YZ, Lee J, Lee D. Current Advances in 3D Bioprinting Technology and Its Applications for Tissue Engineering. Polymers. 2020;12(12):2958.
- Iwanaga S, Arai K, Nakamura M. Inkjet bioprinting. In Essentials of 3D biofabrication and translation 2015 Jan 1 (pp. 61-79). Academic Press.
- Xu T, Baicu C, Aho M, Zile M, Boland T. Fabrication and characterization of bio-engineered cardiac pseudo tissues. Biofabrication. 2009;1(3):035001.
- Bohandy J, Kim BF, Adrian FJ. Metal deposition from a supported metal film using an excimer laser. Journal of Applied Physics. 1986;60(4):1538-9.
- Ramiah P, du Toit LC, Choonara YE, Kondiah PP, Pillay V. Hydrogel-based Bioinks for 3D Bioprinting in Tissue Regeneration. Front. Mater. 2020;7:76.
- Pati F, Jang J, Lee JW, Cho DW. Extrusion bioprinting. In Essentials of 3D biofabrication and translation 2015 Jan 1 (pp. 123-152). Academic Press.